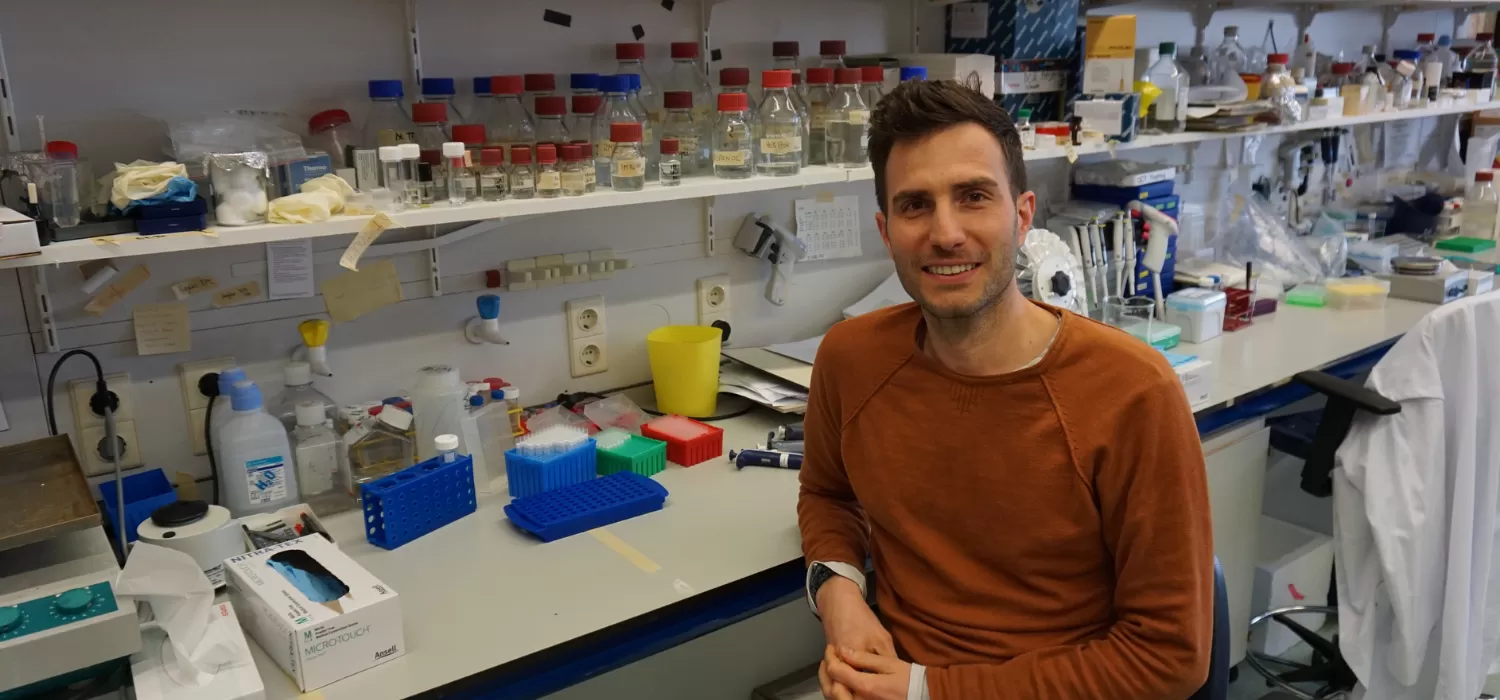
Rett syndrome is caused by mutations to one of the two copies of the MECP2 gene located on the X chromosome. During early embryonic development, female cells randomly inactivate one of their X chromosomes to compensate for the double dosage of having two X chromosomes compared to male cells. This means that sick cells that express the mutated copy of MECP2 also contain a healthy, or wild-type, copy on the silenced X chromosome. Waking up, or reactivating, that silenced wild-type copy of MECP2 is a field that has received increasing scientific attention. This is one of the six strategic curative approaches of the Rett Syndrome Research Trust (RSRT).
I am Hegias Mira-Bontenbal, an assistant professor within Joost Gribnau’s group in the Developmental Biology Department of the Erasmus University Medical Center in Rotterdam, Netherlands. I recently published a paper with my colleagues where we study how the MECP2 gene is reactivated in mouse neuronal stem cells (NSCs). Several years ago, we received funding from RSRT to create a new, more sensitive model system to study MECP2 reactivation. The reasons were several-fold. We needed a new mouse model that allowed us to study the cells that are critical for Rett, neurons. Also, we wanted to create a new model where, instead of using a fluorescent protein to measure X chromosome reactivation (XCR), we could use a bioluminescent protein that is much more sensitive than fluorescence or other available bioluminescent proteins. Bioluminescence is the phenomenon by which fireflies make light! Our final goal was thus to generate a model that could be used to screen for hundreds of thousands of drugs that might reactive the silent healthy copy of MECP2.
We fused the MECP2 gene with a dual reporter system, combining a very sensitive bioluminescent protein, Nanoluciferase, and another fluorescent protein, TdTomato, which, as its name indicates, generates red fluorescent light. Using embryonic stem cells, we generated mice carrying this dual reporter, and through a genetic trick we forced X chromosome inactivation (XCI) towards inactivation of the reporter – which means no cells produced “light.” We isolated NSCs from mouse embryos and kept them in culture to fully characterize them. To show that our system can be used to reactivate MECP2, we used two mechanisms that led to the production of bioluminescence and fluorescence from out NSCs.
As had been published by our collaborators within RSRT in the past, we first reduced the expression of Xist, which is a gene critical for XCI. We believed, as others, that reducing its expression might make the X chromosome more amenable to reactivation. And, secondly, we blocked an enzyme that is important for the maintenance of DNA methylation. DNA methylation is a chemical modification of the DNA base pairs – we call it an epigenetic modification. DNA methylation at positions where genes start is detected by cells as an I-should-be-switched-off signal, leading to gene repression. DNA methylation is thus a mechanism that is used by the cell to lock genes on the X in a repressed mode. By blocking the enzyme that carries this function, we reduce or eliminate DNA methylation leading to gene re-expression.
This is exactly what happened with MECP2! However, this happened in a small percentage of cells and, as expected, to many other genes on the X chromosome. We detected 86 genes among the 2600 X-linked genes that were reactivated along MECP2 and were intrigued by the characteristics that they shared.
Why did these genes reactivate more easily than others? What is it that they share that leads to reactivation of these and not others? We looked at the epigenetic and genetic marks this pool of reactivated genes shares and compared them to non-reactivated genes. We observed that reactivated genes tend to have more active epigenetic marks when they are located on the active X: They are probably more efficient at recruiting factors important for activation. A few small regions on the X also were enriched for reactivated genes compared to other regions. Although we used a blocker of the enzyme that maintains DNA methylation and observed global demethylation, there was no significant demethylation of genes that showed reactivation. This apparently contradictory result can be reconciled with the fact that a small decrease in DNA methylation might be sufficient for factors to bind to genes to reactivate them.
When we focused on the genomic features of reactivated genes, the most significant and surprising correlation was with SINEs, short interspersed nuclear elements. These are small repetitive regions and were more frequently close to reactivated genes than non-reactivated genes. What these SINEs do for reactivation and how that happens mechanistically is still an open question.
Finally, we compared our strategy for XCR with other published techniques to reactivate the X, such as induced pluripotent stem cell reprogramming, and noticed that both pools of reactivated genes overlap substantially, even if reprogramming starts from fibroblasts, a very different cell type compared to neuronal stem cells. This indicates that X chromosome reactivation in different cell types or by different means follows similar paths.
The next steps now are to use these neuronal stem cells, or neurons differentiated from them, to screen for chemical compounds that will lead more efficiently to MECP2 reactivation. What is clear also from this study is that a more targeted approach for MECP2 reactivation is necessary, in order to avoid reactivation of other X-linked genes. Disrupting Xist might also be necessary to reach stable reactivation of MECP2.
We look forward to continuing to contribute to this exciting area of research.
References:
- Mira-Bontenbal, H, et al., Genetic and epigenetic determinantsn of reactivation of Mecp2 and the inactive X chromosome in neural stem cells. Stem Cell Reports, 2022, Vol.17, 693-706.
- Carrette, LLG, et al., A mixed modality approach towards Xi reactivation for Rett syndrome and other X-linked disorders. Proc Natl Acad Sci U S A, 2018. 115(4): p. 668-675.